A range of agriculture practices can impact mycotoxins, including adsorbents that can help prevent absorption of mycotoxins within the animal’s gut.
Support animal health and welfare (mycotoxins)
Mycotoxins are chemical compounds, such as penicillin, that are produced by molds and can have a range of different effects when consumed by humans and/or animals. Penicillin is perhaps the best-known example of a mycotoxin, while there are more than 500 known mycotoxins. Mycotoxins are produced when mold grows on feed or food sources, such as grains.
When feed contaminated with mycotoxins is consumed by animals, it can negatively impact their health by making them more susceptible to challenges from bacteria and viruses. This can lead to higher levels of morbidity and mortality, representing a welfare risk, while also resulting in greater levels of resources required to support the herd or flock. Some key facts related to mycotoxins include:
- More than 97% of feed contains mycotoxins (Weaver et al., 2021).
- Lower levels of multiple mycotoxins can be more harmful than individual mycotoxins (Kipper et al., 2020; Weaver et al., 2020; Andretta et al., 2016).
- A significant element of the damage caused by mycotoxins takes place in the gastrointestinal tract (Alessane-Kpembi et al., 2019; Weaver et al., 2020).
- Mycotoxins can negatively affect an animal’s immune system, resulting in poorer health metrics as well as the failure of vaccine programs (Grenier and Applegate 2013, da Silva et al 2018, Liu et al 2020, Savard et al 2015).
Unfortunately, there is no simple approach to preventing and/or removing mycotoxins from feeds being consumed by humans and animals. Climate plays an important role in the lifecycle of the mold and can therefore significantly influence the amount of mycotoxins produced. As the Earth warms, it is suggested that many temperate areas will face the risk of greater mycotoxin production.
Jouany et al., 2007 and Fumagalli et al., 2021 demonstrate clearly the many steps involved in influencing the level of mycotoxins in feed grains, from agricultural practices such as tillage and crop rotation to the storage and processing of grains. Within this, some important components include:
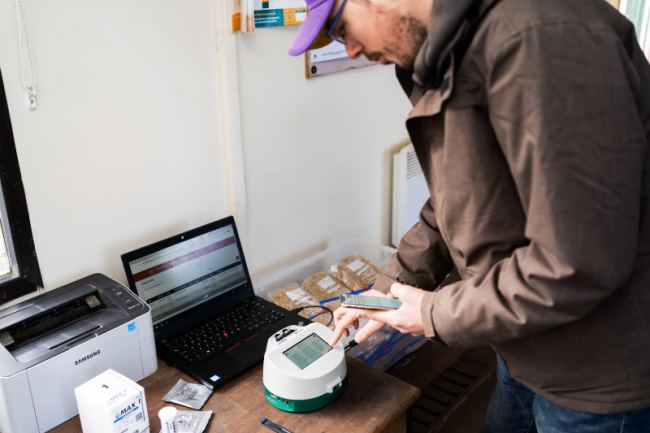
- Tillage is currently seen as one of the steps that can have a significant impact on the reduction of mold growth and mycotoxin production in the subsequent crop (Pfordt et al., 2020). With the move to reduce land tillage, to aid carbon sequestration, an important mycotoxin control point will be lost.
- Grain cleaning/sorting can remove the smaller, damaged, discolored grains and fragments from a given batch. These grains typically contain higher levels of contamination and therefore significant reduction in mycotoxin levels can be achieved, depending on the crop and target mycotoxin.
- Mycotoxin analysis is seen as an important step to determine the extent of contamination of a given batch of grain. This knowledge can help to drive decision-making regarding how that grain is treated and/or used within the feed and food chain.
- Mycotoxin-deactivating technologies offer a final step to help reduce the negative effects of mycotoxins on the animal. Many approaches have been evaluated with the most popular being that of mycotoxin adsorbents, which act as inert molecules that possess the ability to form an interaction with mycotoxins within the animal’s digestive tract, preventing subsequent absorption of the toxin.
References
- Weaver A.C., Weaver D.M., Adams N. and Yiannikouris A. Co-Occurrence of 35 Mycotoxins: A Seven-Year Survey of Corn Grain and Corn Silage in the United States. Toxins 2021, 13, 516. https://doi.org/10.3390/toxins13080516.
- Kipper, M.; Andretta, I.; Ribeiro, A.M.L.; da Silva Pires, P.G.; Franceschina, C.S.; Cardinal, K.M.; de Oliveira Moraes, P.; Schroeder, B. Assessing the implications of mycotoxins on productive efficiency of broilers and growing pigs. Sci. Agric. 2020, 77, e20180236.
- Andretta, I.; Kipper, M.; Hauschild, L.; Lehnen, C.R.; Remus, A.; Melchior, R. Meta-analysis of individual and combined effects of mycotoxins on growing pigs. Sci. Agric. 2016, 73, 328–331.
- Weaver, A.C.; King, W.D.; Verax, M.; Fox, U.; Kudupoje, M.B.; Mathis, G.; Lumpkins, B.; Yiannikouris, A. Impact of Chronic Levels of Naturally Multi-Contaminated Feed with Fusarium Mycotoxins on Broiler Chickens and Evaluation of the Mitigation Properties of Different Titers of Yeast Cell Wall Extract. Toxins 2020, 12, 636.
- Alassane-Kpembi I., Pinton P., Oswald I.P. Effects of Mycotoxins on the Intestine. Toxins (Basel). 2019 Mar; 11(3): 159.
- Fumagalli, F.; Ottoboni, M.; Pinotti, L.; Cheli, F. Integrated Mycotoxin Management System in the Feed Supply Chain: Innovative Approaches. Toxins 2021, 13, 572.
- Jouany J P. Methods for preventing, decontaminating and minimizing the toxicity of mycotoxins in feeds. Animal Feed Science and Technology 137 (2007) 342–362
- Pfordt A., Ramos Romero L., Schiwek S., Karlovsky P., von Tiedemann A. Impact of Environmental Conditions and Agronomic Practices on the Prevalence of Fusarium Species Associated with Ear- and Stalk Rot in Maize. Pathogens 2020, 9, 236
- Grenier, B., and T.J. Applegate. 2013. Modulation of intestinal functions following mycotoxin ingestion: meta-analysis of published experiments in animals. Toxins, 5:396-430.
- Da Silva, E.O., A.P.F.L. Bracarense, and I.P. Oswald. 2018. Mycotoxins and oxidative stress: where are we? World Mycotoxins Journal, 11:113-133.
- Liu, D., L. Ge, J. Su, X. Chen, C. Wang, and K. Huang. 2020. Low-level contamination of deoxynivalenol: a threat from environmental toxins to porcine epidemic diarrhea virus inection. Environment International, 143:105949.
- Savard, C., C. Provost, F. Alvarez, V. Pinilla, N. Music, M. Jacques, C.A. Gagnon, and Y. Chorfi. 2015. Effects of deoxynivalenol (DON) mycotoxin on porcine circovirus type 2 (PCV2) infection. Vet. Microbiol., 176:257-267.
- Liu, Y., Chang, C.C., Marsh, G.M. and Wu, F., 2012. Population attributable risk of aflatoxin-related liver cancer: systematic review and meta-analysis. European Journal of Cancer 48: 2125-2136.
- Shuaib, F.M., Jolly, P.E., Ehiri, J.E., Yatich, N., Jiang, Y., Funkhouser, E., Person, S.D., Wilson, C., Ellis, W.O., Wang, J.S. and Williams, J.H., 2010. Association between birth outcomes and aflatoxin B1 biomarker blood levels in pregnant women in Kumasi Ghana. Tropical Medicine and International Health 15: 160-167.
- Mahdavi, R., Nikniaz, L., Arefhosseini, S.R. and Vahed Jabbari, M., 2010. Determination of aflatoxin M1 in breast milk samples in Tabriz-Iran. Maternal Child Health Journal 14: 141-145.
- Sadeghi, N., Oveisi, M., Jannat, B., Hajimahmoodi, M., Bonyani, H., and Jannat, F., 2009. Incidence of aflatoxin M1 in human breast milk in Tehran, Iran. Food Control 20: 75-78.
- Wu, F. 2015. Global impacts of aflatoxin in maize: trade and human health. World Mycotoxin J. 8:137-142.